A triangle is one of the basic shapes of geometry: a polygon with three corners or vertices and three sides or edges which are line segments. A triangle with vertices A, B, and C is denoted
ABC.
In Euclidean geometry any three non-collinear points determine a unique triangle and a unique plane (i.e. a two-di! mensional Euclidean space).
Types of triangles
By relative lengths of sides
Triangles can be classified according to the relative lengths of their sides:
- In an equilateral triangle, all sides are the same length. An equilateral triangle is also a regular polygon with all angles equal to 60°.[1]
- In an isosceles tri! angle, two sides are equal in length. (Traditionally, only two sides equal, but sometimes at least two.)[2] An isosceles triangle also has two equal angles: the angles opposite the two equal sides.
- In a scalene triangle, all sides and internal angles are different from one another.[3]
 |
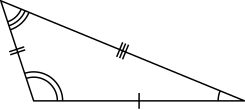 |
Equilateral | Isosceles | Scalene |
By internal angles
Triangles can also be classified according to their internal angles, mea! sured here in degrees.
- A right triangle (or right-angled triangle, formerly called a rectangled triangle) has one of its internal angles equal to 90° (a right angle). The side opposite to the right angle is the hypotenuse; it is the longest side in the right triangle. The other two sides are the legs or catheti[citation needed] (singular: cathetus) of the triangle. Right triangles obey the Pythagorean theorem: the sum of the squares of the two legs is equal to the square of the hypotenuse: a2 + b2 = c2, where a and b are the lengths of the legs and c is the length of the hypotenuse. Special right triangles are right triangles with additional properties that make calculations involving them easier.
- Triangles that do not have a 90° internal angle are called oblique triangles.
- A triangle that has all the internal angles smaller than 90° is an acute triangle or acute-angled triangle.
- A triangle that has one angle larger than 90° is an obtuse triangle or obtuse-angled triangle.
- An equilateral triangle! b> is an acute triangle with all three internal angles equal to 60°.
 |  |  |
Right Obtuse | Acute |
|  |
| Oblique |
[edit] Basic facts
Triangles are assumed to be two-dimensional plane figures, unless the context provides otherwise (see Non-planar triangles, below). In rigorous treatments, a triangle is therefore called a 2-simplex (see also Polytope). Elementary facts about triangles were presented by Euclid in books 1–4 of his Elements, around 300 BCE.
A triangle, showing exterior angle d
The internal angles of a triangle in Euclidean space always add up to 180 degrees. This allows determination of the third angle of any triangle as soon as two angles are known. An external angle, or exterior angle, of a triangle is an angle that is adjacent and supplementary to an internal angle. Any external angle of any triangle is equal to the sum of the two internal angles that it is not adjacent to; this is the exterior angle theorem. The three external angles (one for each vertex) of any triangle add up to 360 degrees.[4]
The sum of the lengths of any two sides of a triangle always exceeds the length of the third side, a principle known as the triangle inequality.[5]
Two triangles are said to be similar if and only if each internal angle of one triangle is equal to an internal angle of the other.[6] In this case, all sides of one triangle are in equal proportion to sides of the other triangle.
A few basic postulates and theorems about similar triangles:
- If two corresponding internal angles of two triangles are equal, the triangles are similar.
- If two corresponding sides of two triangles are in proportion, and their included angles are equal, then the triangles are similar. (The included angle for any two sides of a polygon is the internal angle between those two sides.)
- If three corresponding sides of two triangles are in proportion, then the triangles are similar.[7]
Two triangles that are congruent have exactly the same size and shape:[8] all pairs of corresponding internal angles are equal in size, and all pairs of corresponding sides are equal in length. (This is a total of six equalities, but three are often sufficient to prove congruence.)
Some sufficient conditions for a pair of triangles to be congruent (from basic postulates and theorems of Euclid):
- SAS Postulate: Two sides and the included angle in a triangle are equal to two! sides and the included angle in the other triangle.
- A! SA Postu late: Two internal angles and the included side in a triangle are equal to those in the other triangle. (The included side for a pair of angles is the side between them.)
- SSS Postulate: Each side of a triangle is equal in length to a side of the other triangle.
- AAS Theorem: Two angles and a corresponding non-included side in a triangle are equal to those in the other triangle.
- Hypotenuse-Leg (HL) Theorem: The hypotenuse and a leg in a right triangle are equal to those in the other right triangle.
- Hypotenuse-Angle Theorem: The hypotenuse and an acute angle in one right triangle are equal to those in the other right triangle.
An important case:
- Side-Side-Angle (or Angle-Side-Side) condition: If two sides and a corresponding non-included angle of a triangle are equal to those in another, then this is not sufficient to prove congru! ence; but if the non-included angle is obtuse or a right angle, or the side opposite it is the longest side, or the triangles have corresponding right angles, then the triangles are congruent. The Side-Side-Angle condition does not by itself guarantee that the triangles are congruent because one triangle could be obtuse-angled and the other acute-angled.
Using right triangles and the concept of similarity, the trigonometric functions sine and cosine can be defined. These are functions of an angle which are investigated in trigonometry.
A central theorem is the Pythagorean theorem, which states in any right triangle, the square of the length of the hypotenuse equals the sum of the squares of the lengths of the two other sides. If the hypotenuse has length c, and the legs have lengths a and b, then the theorem states that

The converse is true: if the lengths of the sides of a triangle satisfy the above equation, then the triangle has a right angle opposite side c.
Some other facts about right triangles:

- If the legs of a right triangle are equal, then the angles opposite the legs are equal, acute, and complementary; each is therefore 45 degrees. By the Pythagorean theorem, the length of the hypotenuse is the length of a leg times √2.
- In a right triangle with acute angles measuring 30 and 60 degrees, the hypoten! use is twice the length of the shorter side, and twice the len! gth divi ded by √3 for the longer side.
For all triangles, angles and sides are related by the law of cosines and law of sines (also called the cosine rule and sine rule).
Points, lines and circles associated with a triangle
There are hundreds of different constructions that find a special point associated with (and often inside) a triangle, satisfying some unique property: see the references section for a catalogue of them. Often they are constructed by finding three lines associated in a symmetrical way with the three sides (or vertices) and then proving that the three lines mee! t in a single point: an important tool for proving the existence of these is Ceva's theorem, which gives a criterion for determining when three such lines are concurrent. Similarly, lines associated with a triangle are often constructed by proving that three symmetrically constructed points are collinear: here Menelaus' theorem gives a useful general criterion. In this section just a few of the most commonly-encountered constructions are explained.
The
circumcenter is the center of a circle passing through the three vertices of the triangle.
A perpendicular bisector of a triangle is a straight line passing through the midpoint of a side and being perpendicular to it, i.e. forming a right angle wit! h it. The three perpendicular bisectors meet in a single point, the triangle's circumcenter; this point is the center of the circumcircle, the circle passing through all three vertices. The diameter of this circle can be found from the law of sines stated above.
Thales' theorem implies that if the circumcenter is located on one side of the triangle, then the opposite angle is a right one. More is true: if the circumcenter is located inside the triangle, then the triangle is acute; if the circumcenter is located outside the triangle, then the triangle is obtuse.
An altitude of a triangle is a straight line through a vertex and perpendicular to (i.e. forming a right angle with) the opposite side. This opposite side is called the ba! se of the altitude, and the point where the altitude intersects the base (or its extension) is called the foot of the altitude. The length of the altitude is the distance between the base and the vertex. The three altitudes intersect in a single point, called the orthocenter of the triangle. The orthocenter lies inside the triangle if and only if the triangle is acute. The three vertices together with the orthocenter are said to form an orthocentric system.
The intersection of the angle bisectors finds the center of the
incircle.
An angle bisector of a triangle is a straight line through a vertex which cuts the corresponding angle in half. The three angle bisectors intersect in a single point, the incenter, the center of the triangle's incircle. The inc! ircle is the circle which lies inside the triangle and touches all three sides. There are three other important circles, the excircles; they lie outside the triangle and touch one side as well as the extensions of the other two. The centers of the in- and excircles form an orthocentric system.
The intersection of the medians is the
centroid.
A median of a triangle is a straight line through a vertex and the midpoint of the opposite side, and divides the triangle into two equal areas. The three medians intersect in a single point, the triangle's centroid. The centroid of a stiff triangular object (cut out of a thin sheet of uniform density) is also its center of gravit! y: the object can be balanced on its centroid. The centroid cuts every median in the ratio 2:1, i.e. the distance between a vertex and the centroid is twice the distance between the centroid and the midpoint of the opposite side.
Nine-point circle demonstrates a symmetry where six points lie on the edge of the triangle.
The midpoints of the three sides and the feet of the three altitudes all lie on a single circle, the triangle's nine-point circle. The remaining three points for which it is named are the midpoints of the portion of altitude between the vertices and the orthocenter. The radius of the nine-point circle is half that of the circumcircle. It touches the incircle (at the Feuerbach point) and the three excircles.
Euler's line is a straight line through the centroid (orange), orthocenter (blue), circumcenter (green) and center of the nine-point circle (red).
The centroid (yellow), or! thocenter (blue), circumcenter (green) and barycenter of the n! ine-poin t circle (red point) all lie on a single line, known as Euler's line (red line). The center of the nine-point circle lies at the midpoint between the orthocenter and the circumcenter, and the distance between the centroid and the circumcenter is half that between the centroid and the orthocenter.
The center of the incircle is not in general located on Euler's line.
If one reflects a median at the angle bisector that passes through the same vertex, one obtains a symmedian. The three symmedians intersect in a single point, the symmedian point of the triangle.
Computing the area of a triangle
The area of a triangle can be demonstrated as half of the area of a
paralellogram which has the same base length and he! ight.
Calculating the area of a triangle is an elementary problem encountered often in many different situations. The best known and simplest formula is:

where A is area, b is the length of the base of the triangle, and h is the height or altitude of the triangle. The term 'base' denotes any side, and 'height' denotes the length of a perpendicular from the point opposite the side onto the side itself.
Although simple, this formula is only useful if the height can be readily found. For example, the surveyor of a triangular field measures the length of each side, and can find the area from his results without having to construct a 'height'. Various methods may be used in practice, depending on what is k! nown about the triangle. The following is a selection of frequently used formulae for the area of a triangle.[9]
Using vectors
The area of a parallelogram can be calculated using vectors. Let vectors AB and AC point respectively from A to B and from A to C. The area of parallelogram ABDC is then
, which is the magnitude of the cross product of vectors AB! and AC.
is equal to
, where h represents the altitude h as a vector.
The area of triangle ABC is half of this, or
.
The area of triangle ABC can also be expressed in terms of dot products as follows:

Applying trigonometry to find the altitude h.
Using trigonometry
The height of a triangle can be found through an application of trigonometry. Using the labelling as in the image on the left, the altitude is h = a sin γ. Substituting this in the formula ! S = ½bh derived above, the area of the triangl! e can be expressed as:

(where α is the interior angle at A, β is the interior angle at B, and γ is the interior angle at C).
Furthermore, since sin α = sin (Ï - α) = sin (β + γ), and similarly for the other two angles:

Using coordinates
If vertex A is located at the origin (0, 0) of a Cartesian coordinate system and the coordinates of the other two vertices are given by B = (xB, yB) and C = (xC, yC), then the area S can be computed as ½ times the absolute value of the determinant

For three general vertices, the equation is:


In three dimensions, the area of a general triangle {A = (xA, yA, zA), B = (xB, yB, zB) and C = (xC, yC, zC)} is the Pythagorean sum of the areas of the respective projections on the three principal planes (i.e. x = 0, y = 0 and z = 0):

Using Heron's formula
The shape of the triangle is determined by the lengths of the sides alone. Therefore the area S also can be derived from the lengths of the sides. By Heron's formula:

where s = ½ (a + b + c) is the semiperimeter! b>, or half of the triangle's perimeter.
Three equivale! nt ways of writing Heron's formula are



Computing the sides and angles
In general, there are various accepted methods of calculating the length of a side or the size of an angle. Whilst certain methods may be suited to calculating values of a right-angled triangle, others ! may be required in more complex situations.
Trigonometric ratios in right triangles
A right triangle always includes a 90° (Ï/2 radians) angle, here labeled C. Angles A and B may vary. Trigonometric functions specify the relationships among side lengths and interior angles of a right triangle. In right triangles, the trigonometric ratios of sine, cosine and tangent can be used to find unknown angles and the lengths of unknown sides. The sides of the triangle are known as follows:
- The hypotenuse is the side opposite the right ! angle, or defined as the longest side of a right-angled triangle, in this case h.
- The opposite side is the side opposite to the angle we are interested in, in this case a.
- The adjacent side is the side that is in contact with the angle we are interested in and the right angle, hence its name. In this case the adjacent side is b.
Sine, cosine and tangent
The sine of an angle is the ratio of the length of the opposite side to the length of the hypotenuse. In our case

Note that this ratio does not depend on the particular right triangle chosen, as ! long as it contains the angle A, since all those triang! les are similar.
The cosine of an angle is the ratio of the length of the adjacent side to the length of the hypotenuse. In our case

The tangent of an angle is the ratio of the length of the opposite side to the length of the adjacent side. In our case
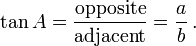
The acronym "SOHCAHTOA" is a useful mnemonic! for these ratios.
Inverse functions
The inverse trigonometric functions can be used to calculate the internal angles for a right angled triangle with the length of any two sides.
Arcsin can be used to calculate an angle from the length of the opposite side and the length of the hypotenuse.

Arccos can be used to calculate an angle from the length of the adjacent side and the length of the hypontenuse.
![\theta = \arccos \left( \frac{\text{adjacent}}{\text{hypotenuse}} \right)]()
Arctan can be used to calculate an angle from the length of the opposite side and the length of the adjacent side.

The sine and cosine rules
A triangle with sides of length a, b and c and angles of α, β and γ respectively.
The law of sines, or sine rule[10], states that the ratio of the length of side a to the! sine of its corresponding angle α is eq ual to the ratio of the length of side b to the sine of its corresponding angle β.

The law of cosines, or cosine rule, connects the length of an unknown side of a triangle to the length of the other sides and the angle opposite to the unknown side. As per the law:
For a triangle with length of sides a, b, c and angles of α, β, γ respectively, given two known lengths of a triangle a and b, and the angle between the two known sides γ (or the angle opposite to the unknown side c), to calculate the third side c, the following formula can be used:

Non-planar triangles
A non-planar triangle is a triangle which is not contained in a (flat) plane. Examples of non-planar triangles in non-Euclidean geometries are spherical triangles in spherical geometry and hyperbolic triangles in hyperbolic geometry.
While the internal angles in planar triangles always add up to 180°, a hyperbolic triangle has angles that add up to less than 180°, and a spherical triangle has angles that add up to more than 180°. A hyperbolic triangle can be obtained by drawing on a negatively-curved surface, such as a saddle surface, and a spherical triangle can be obtained by drawing on a positively-curved surface such as a sphere. Thus, if one draws a giant triangle on the surface of the Earth, one will find that the sum of its angles is greater than 180°. It is possible! to draw a triangle on a sphere such that each of its internal angles is equal to 90°, adding up to a total of 270°.
facts about triangles